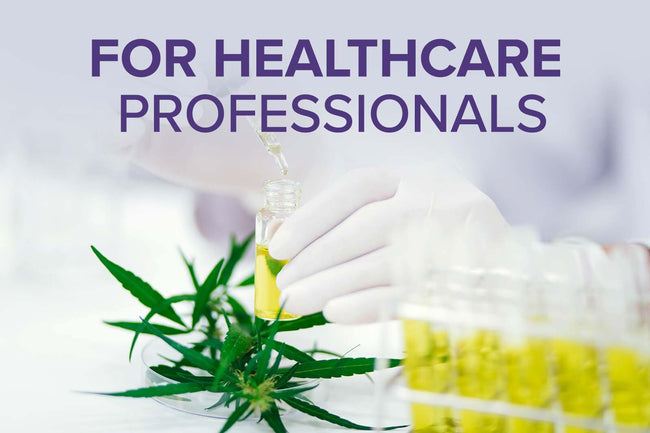
CBD Research in Pharmacology Research & Perspectives
Diversity of molecular targets and signalling pathways for CBD
Douglas L. de Almeida,Lakshmi A. Devi
Abstract
Cannabidiol (CBD) is the second most abundant component of the Cannabis plant and is known to have effects distinct from Δ9-tetrahydrocannabinol (THC). Many studies that examined the behavioral effects of CBD concluded that it lacks the psychotomimetic effects attributed to THC. However, CBD was shown to have a broad spectrum of effects on several conditions such as anxiety, inflammation, neuropathic pain, and epilepsy. It is currently thought that CBD engages different targets and hence CBD’s effects are thought to be due to multiple molecular mechanisms of action. A well-accepted set of targets include GPCRs and ion channels, with the serotonin 5-HT1A receptor and the transient receptor potential cation channel TRPV1 channel being the two main targets. CBD has also been thought to target G protein-coupled receptors (GPCRs) such as cannabinoid and opioid receptors. Other studies have suggested a role for additional GPCRs and ion channels as targets of CBD. Currently, the clinical efficacy of CBD is not completely understood. Evidence derived from randomized clinical trials, in vitro and in vivo models and real-world observations support the use of CBD as a drug treatment option for anxiety, neuropathy, and many other conditions. Hence an understanding of the current status of the field as it relates to the targets for CBD is of great interest so, in this review, we include findings from recent studies that highlight these main targets.
Abbreviations
2-AG - 2 Arachidonoylglycerol
5-HT1A - 5-hydroxytryptamine 1A receptor
[3H]8-OH-DPAT - 7-(Dipropylamino)-5,6,7,8-tetrahydronaphthalen-1-ol
AEA - Anandamide
AM 251 - 1-(2,4-Dichlorophenyl)-5-(4-iodophenyl)-4-methyl-N-(1-piperidyl)pyrazole-3-carboxamide
AM 630 - 1-[2-(Morpholin-4-yl)ethyl]-2-methyl-3-(4-methoxybenzoyl)-6-iodoindole
BHK - Baby hamster kidney cell line
BRET - Bioluminescence resonance energy transfer
CB1 - Cannabinoid receptor 1
CB2 - Cannabinoid receptor 2
CBD - Cannabidiol
CHO - Chinese hamster ovary cell line
CP 55940 - 2-[(1R,2R,5R)-5-Hydroxy-2-(3-hydroxypropyl)cyclohexyl]-5-(2-methyloctan-2-yl)phenol
DAMGO - [D-Ala2, N-MePhe4, Gly-ol]-enkephalin
dlPAG - dorsolateral periaqueductal gray
DPCPX - 8-Cyclopentyl-1,3-dipropylxanthine
EEG - Electroencephalogram
EMT - Endocannabinoid membrane transporter
FAAH - Fatty acid amide hydrolase
GPCR - G-protein coupled receptor
GPR55 - G-protein receptor 55
GTPγS - Guanosine triphosphate gamma S
HEK 293 - human embryonic kidney 293 cell
HU 210 - (6aR,10aR)-9-(hydroxymethyl)-6,6-dimethyl-3-(2-methyloctan-2-yl)-6H,6aH,7H,10H,10aH-benzo[c]isochromen-1-ol
LPI - Lysophosphatidylinositol
M3 - Muscarinic receptor 3
MAGL - Monoacyl glycerol lipase
MIA - Monoiodoacetate
MTT - 3-(4,5-dimethylthiazol-2-yl)-2,5-diphenyltetrazolium bromide
OBX - olfactory bulbectomy mouse model of depression
PLA - Phospholipase A
PPARγ - peroxisome proliferator-activated receptor gamma
PTZ - pentylenetetrazole
rCBF - regional cerebral blood flow
RVM - rostroventral medulla
SB 366791 - 4'-Chloro-3-methoxycinnamanilide
SR 141716 - N-(Piperidin-1-yl)-5-(4-chlorophenyl)-1-(2,4-dichlorophenyl)-4-methyl-1H-pyrazole-3-carboxamide hydrochloride
SR 144528 - 5-(4-Chloro-3-methylphenyl)-1-[(4-methylphenyl)methyl]-N-[(1S,2S,4R)-1,3,3-trimethylbicyclo[2.2.1]hept-2-yl]-1H-pyrazole-3-carboxamide
THC - Δ9-tetrahydrocanabinol
TRPA1 - transient receptor potential ankyrin 1
TRPV1 - transient receptor potential vaniloid 1
vmPAG - ventromedial periaqueductal gray
VR1 - Vanilioid receptor 1
WAY 100635 - N-[2-[4-(2-Methoxyphenyl)-1-piperazinyl]ethyl]-N-2-pyridinylcyclohexanecarboxamide maleate
WIN 55212 - (R)-(+)-[2,3-Dihydro-5-methyl-3-(4-morpholinylmethyl)pyrrolo[1,2,3-de]-1,4-benzoxazin-6-yl]-1-naphthalenylmethanone mesylate
1. INTRODUCTION
The plant, Cannabis sativa, has been used for recreational purposes for more than 4000 years. Over 60 compounds have been identified in the plant, of which the two major pharmacologically active components are –9 tetrahydrocannabinol (Δ9-THC) and cannabidiol (CBD).1
CBD has been shown to alter several body functions and neuronal activity. For example, CBD has been reported to improve motor activity,2 affect depression,3 exhibit antitumorigenic activity in vitro and in vivo,4 anti-inflammatory effects through reduction of pro-inflammatory cytokine synthesis,5 ameliorate lipid and glycemic parameters in Type 2 Diabetes,6 and to reduce markers of inflammation in pancreas microcirculation in a Type 1 Diabetes mice model.7 An interesting study in humans showed that a single high dose of CBD decreased neuronal activity in limbic and paralimbic areas of the brain leading the investigators to conclude that CBD has anxiolytic effects.8 These results were in accordance with a study9 reporting that a similar high dose of CBD was optimally effective in inducing anxiolytic effects in a simulated public speaking test. Finally, a number of studies have reported that CBD can reduce the anxiety and psychosis-like effects seen after THC administration, and attenuate the emotional and reward processing impairments associated with a single high dose of THC (reviewed by (Freeman et al, 201910).
THC and CBD show antioxidant properties and these are thought to be due to a shared chemical structure. The hydroxyl groups and double bonds present in both molecules, contribute to increase their highest occupied molecular orbital (HOMO) value; higher HOMO values indicate a higher ability of the molecule to donate an electron, making THC and CBD powerful antioxidant molecules.11 Moreover studies using cyclic voltammetry and a fenton reaction-based system showed that CBD could reduce hyperoxide toxicity in neurons stimulated with glutamate. The antioxidant effect of CBD, evaluated in rat cortical neuron cultures, was not affected by the presence of 500 nmol/L of the selective CB1 cannabinoid receptor antagonist SR-141716A in an in vitro preparation of ischemic injury and was higher than the effect of other antioxidants such as α-tocopherol and ascorbate in AMPA/kainate receptor toxicity assays.12 In agreement with these findings, Hacke et al,13 reported that the antioxidant activity of THC and CBD in pure and mixed solutions was comparable to that of well-known antioxidants such as ascorbic acid (AA), resveratrol (Resv), and (-)-epigallocatechin-3-gallate (EGCG).
Most of the effects associated with CBD are believed to be mediated through its agonistic properties at 5-HT1A14 and TRPV115 receptors (Figure 1). Furthermore, it has been argued that through different mechanisms of action, CBD can modulate neuronal activity in the dorsal periaqueductal gray, bed nucleus of the stria terminalis and medial prefrontal cortex to exert anxiolytic effects. This has been extensively reviewed by Campos et al (2012).16 In addition to summarizing the targets for CBD described earlier, in the present review we include findings from recent studies to highlight the current status of the field.
Figure 1
Multiple molecular targets for CBD – Cannabidiol has multiple molecular targets within the cell. It behaves as an antagonist for cannabinoid CB1 and CB2 receptors; however, some of the cannabinoid-mediated effects attributed to CBD may be due to its ability to inhibit endocannabinoid degradation through the FAAH enzyme. This, in turn, increases endocannabinoid levels causing receptor activation, mainly by anandamide. The full agonism at 5-HT1A serotonin receptors and TRPV1 channels is responsible for the anxiolytic and analgesic effects in animals. Partial agonism at D2 dopamine receptors might account for the effects of CBD on emotional memory processing by the ventral hippocampus. Full agonism at adenosine A1 receptors might have beneficial effects on cardiac arrythmias and ischemia/reperfusion lesions in the myocardium. The negative allosteric modulation of MOR is an important CBD feature in controlling opioid drug abuse and relapse. Agonism of intracellular PPARγ receptors causes changes in gene transcription and is responsible for the positive effect of CBD on glucose and fatty acid metabolism both in animals and in humans. CBD has an overall inhibitory effect on sodium and calcium channels exerting a modulatory effect on membrane electrical potential; this would suggest CBD as a potential therapeutic for the treatment of epilepsy. CBD, cannabidiol; A1, Adenosine receptor 1; ENT, equilibrative nucleotide transporter; AEA, anandamide; 2-AG, 2-arachidonoylethanolamide; EMT, endocannabinoid membrane transporter; 5-HT1A, 5-hidroxytriptamine 1A receptor; TRPV1, transient receptor potential vanilloid 1; D2, dopamine receptor 2; GPR55, G protein coupled receptor 55; MOR, µ opioid receptor; PPARγ, peroxisome proliferator-activated receptor gamma; CB1, cannabinoid receptor 1; CB2, cannabinoid receptor 2
2. CANNABINOID SYSTEM
Early studies exploring the targets for CBD focused on the cannabinoid receptor system. This system is composed of two main receptors CB1 and CB2, their endogenous ligands (mainly arachidonoylethanolamide – AEA; and 2-arachidonoylglycerol - 2-AG) and the enzymes responsible for endocannabinoid synthesis, reuptake and degradation (Fatty Acid Amide Hydrolase and Mono Acyl Glycerol Lipase – FAAH, and MAGL respectively).17 CB1 receptors are mainly distributed in the central nervous system while the CB2 receptors are mainly present in peripheral nerve terminals and immune cells, although evidence shows that this receptor is expressed in the brain stem (for a more detailed review, see Di Marzo et al, 200417). Unlike other neurotransmitters that are synthesized and stored in vesicles, endocannabinoids are synthesized on demand, after neuronal activation, in postsynaptic terminals in a Ca2+-dependent manner and activate presynaptic Gi/0 cannabinoid receptors. This molecular machinery represents a retrograde signaling mechanism model responsible for long term depression of stimulatory glutamatergic neurons, and control of short-term and long-term neuronal plasticity.18
Initial studies examining the molecular pharmacological properties of CBD reported that it targets the cannabinoid receptor system. CBD was found to displace binding of radiolabeled CB1 and CB2 cannabinoid receptor agonists ([3H]CP55940 and [3H] R-(+)-WIN55212, respectively) with a Ki value of 120.2 nmol/L for the CB1 receptor and 100 nmol/L for the CB2 receptor19 (reviewed in Pertwee, RG; 200820). Furthermore, CBD reduced the efficacy and potency of signaling by 2-AG and Δ9-THC in cells heterologously (HEK 293A) or endogenously (STHdhQ7/Q7) expressing CB1 receptors.21 CBD was also found to display antagonistic activity at CB1 and CB2 cannabinoid receptors since it produced rightward shifts in dose response curves with CP55940- and R-(+)-WIN55212 in G protein activity assays with membranes from CHO cells expressing these receptors and from mouse brain.22 In experiments performed with brain membranes, the mean apparent KB values of CBD for antagonism of CP55940- and R-(___)-WIN55212-induced stimulation of [35S]GTPγS binding to these membranes are 79 and 138 nmol/L, respectively, both well below the Ki value of CBD for its displacement of [3H]CP55940 from specific binding sites on these membranes.20 Finally, Pertwee et al,23 showed that CBD exhibited antagonistic activity at cannabinoid receptors on electrically evoked contractions of the mouse isolated vas deferens.
It is important to point out that the modulatory effects CBD exerts over the psychotomimetic actions of THC in the central nervous system10 might come from its negative allosteric modulation of CB1 receptors, as reported by Laprairie et al.21 To further reinforce this molecular relation between THC and CBD, Hudson et al24 showed that CBD reverses THC associated side-effects due to inhibition of THC-mediated ERK phosphorylation in the ventral hippocampus (vHipp) of Sprague Dawley rats, as assessed by the western blot technique. Furthermore, using the open field test, the authors observed differential effects of THC vs CBD on anxiety-like behaviours. Coadministration of THC and CBD induced a significant anxiolytic effect, with rats spending significantly greater time in the center zone in relation to vehicle and THC treated groups, suggesting that intra-vHipp THC/CBD coadministration produces opposite effects on anxiety relative to THC. Moreover blockade of MEK1–2 signaling dose dependently blocks the anxiogenic effects of intra-vHipp THC, consistent with its ability to prevent intra-vHipp pERK1–2 activation.
In addition to cannabinoid receptors, CBD has also been shown to target the endocannabinoid system. CBD was found to inhibit the activity of FAAH, a major enzyme involved in anandamide hydrolysis.15 Furthermore, the ability of CBD to inhibit AEA hydrolysis and reuptake causes an increase in the concentration of available endogenous cannabinoids to bind their respective receptors. These data are corroborated by studies by Maione et al25 that detected increases in 2-AG in the ventromedial PAG (assessed by microdialysis) after a 3 nmol CBD microinjection. Since anandamide is the main endogenous CB1 receptor agonist, this suggests an indirect effect of CBD on cannabinoid receptors due to increases in endogenous AEA levels. This could explain some of the cannabinoid-mediated effects attributed to CBD, even though it has been otherwise shown to be a cannabinoid receptor antagonist.22 For example, CBD was shown to reduce inflammation in a rat model of osteoarthritis,26 in a model of allergic contact dermatitis,27 and in a model of experimentally inflamed explant human colonic tissue28; the anti-inflammatory effects could be blocked by selective CB2 receptor antagonists. In addition, a study by Maione et al,25 showed that CBD injected into the ventrolateral PAG induced antinociception that could be blocked by the selective CB1 receptor antagonist, AM 251. These studies suggest agonistic activities for CBD that could be due to its ability to inhibit FAAH activity and thereby increase anandamide levels. An interesting observation was made by Massi et al,29 who found that in vivo treatment of nude mice with CBD markedly enhanced the activity of the FAAH enzyme and reduced levels of AEA in tumor samples. These differential effects of CBD on FAAH enzyme activity could be due to differences in tissue levels of FAAH or in the different methods of assessing enzymatic activity.
A study examined the effect of CBD following direct microinjection into the ventrolateral PAG and found that this led to a reduction in the firing rate of ON and OFF cells on the rostral ventromedial medulla (RVM), and its immediate downstream neuronal circuit involved in descending pain modulation.25 These effects were maximal with 3 nmol CBD and were antagonized by selective antagonists of cannabinoid CB1 (AM 251), adenosine A1 (DPCPX), and TRPA1 (AP18), but not TRPV1 receptors (5′-iodo-resiniferatoxin).25 These results support the idea that CBD functions by engaging multiple targets (Table 1).
TABLE 1. Overview of CBD molecular targets
Target |
CBD Effect |
Experiments/Results |
References |
CB1 receptor |
Antagonist |
CBD decreases THC and 2-AG potencies in a GTPγS binding assay in mouse brain membranes |
[22] |
Negative allosteric modulator |
CBD allosterically reduces CB1 receptor signaling in HEK 293A cells |
[21] |
|
CB2 receptor |
Antagonist |
CBD decreases the potency of the receptor agonist, WIN55212, in a GTPγS assay with membranes from CHO cells overexpressing CB2 receptors |
[22] |
FAAH |
Inhibitor |
CBD inhibits [14C]-AEA hydrolysis (IC50 < 100 µmol/L) in N18TG2 cell membrane preparations |
[15] |
GPR55 |
Antagonist |
CBD decreases the potency of the agonist, CP55940, at nmol/L concentrations in a GTPγS assay with membranes from cells overexpressing GPR55 |
[82] |
5-HT1A |
Agonist |
CBD displaces [3H]8-OH-DPAT binding and increases G protein activity in CHO cells overexpressing the human 5-HT1A receptor |
[14] |
Anxiolytic-like properties |
CBD increases the distance travelled in an open field test in a mouse model of depression (OBX); this is blocked by a selective 5-HT1A receptor antagonist, WAY100635. CBD increases sucrose consumption in the sucrose preference test, and glutamate release as assessed by microdialysis studies |
[83] |
|
Analgesia |
Reversal of CBD-mediated analgesia by a selective 5-HT1A receptor antagonist, WAY 100135, in a Von Frey filament test |
[36] |
|
Dopamine D2 receptor |
Partial agonist |
CBD inhibits radiolabeled domperidone binding to D2 receptors with dissociation constants of 11 nmol/L at dopamine D2High receptors and 2800 nmol/L at dopamine D2Low receptors in rat striatal membranes |
[38] |
Adenosine A1 receptor |
Agonist |
CBD induces antiarrhythmic effects against I/R-induced arrhythmias in rats; this is blocked by the adenosine A1 receptor antagonist DPCPX |
[45] |
Adenosine A2A receptor |
Agonist |
Treatment with CBD (1 mg/kg) singinficantly reduces TNFα in mice challenged with LPS; this is blocked by pre-treatment with the A2A adenosie receptor antagonist ZM 241385 (10 mg/kg, i.p.) |
[43] |
MOR and DOR |
Allosteric modulator |
CBD accelerates [3H]DAMGO dissociation from MOR and [3H]-NTI from DOR induced by 10 μmol/L naloxone or 10 µmol/L naltrindole, respectively, in cerebral cortical tissue from male Wistar rats (assessed by kinetic binding studies) |
[47] |
TRPV1 |
Agonist |
CBD increases cytosolic calcium levels to the same extent as the full agonist capsaicin in HEK 293 cells overexpressing the human TRVR1 receptor. |
[15] |
CBD reduces leaver pressing in a cocaine self-administration test; this is blunted by capsazepine, a TRPV1 receptor antagonist |
[62] |
||
Sodium channels |
Inhibition |
CBD inhibits hNav1.1-1.7 currents (IC50 of 1.9–3.8 μmol/L). Voltage-clamp electrophysiology in HEK-293 cells and iPSC neurons shows that CBD preferentially stabilizes inactivated Nav channel states |
[63] |
Calcium channels |
Inhibition of L-type channels |
Patch-clamp techniques show that CBD inhibits L-type Ca2+ channels (IC50 of 0.1 µmol/L) in rat myocytes. |
[65] |
Bidirectional effect on Ca2+ levels |
Mitochondrion-specific Ca2+ sensor, Rhod-FF, shows that CBD reduces [Ca2+]i levels under high excitability conditions but causes an increase under basal conditions in hippocampal primary neuronal cultures |
[66] |
|
PPARγ receptor |
Agonist |
CBD induces reactive gliosis in rat primary astroglial cultures; this is significantly blunted by a selective antagonist of PPARγ receptors, GW9662 |
[72] |
Anti-inflammatory |
CBD reduces leukocyte rolling and adhesion to the endothelium in a MIA-injected model of inflammation in rats |
[26] |
|
Antioxidant |
CBD reduces hyperoxide toxicity in neurons stimulated with glutamate (evaluated by cyclic voltammetry and a fenton reaction-based system); this is not altered by cannabinoid receptor antagonists |
[12] |
3. GPR55
GPR55 has been proposed to be a third cannabinoid receptor responsible for some effects attributed to cannabinoids that do not seem to be mediated through CB1 or CB2 receptors.30 Ryberg et al22 showed that cannabinoid receptor agonists such as CP55940, HU210, and Δ9-THC can bind to and signal in heterologous cells expressing FLAG-tagged human GPR55. Like its activity at CB1 or CB2 receptors,22 CBD appears to function as a GPR55 antagonist.31 CBD decreases the potency of the agonist, CP55940, at nmol/L concentrations in a GTPγS assay with membranes from cells overexpressing GPR55.30
To examine the in vivo effects, a synthetic regioisomer of cannabidiol named abnormal-cannabidiol (Abn-CBD), was used; administration of Abn-CBD produced vasodilator effects, reduced blood pressure, did not have any psychotomimetic effects,32 and showed that it could be a powerful tool to manage some of Parkinson’s disease symptoms.33 Also, Abn-CBD has an anti-cataleptic effect that is blocked by CBD confirming the agonist-antagonist activities of these two molecules at GPR55.33
4. 5-HT1A RECEPTORS
One of the main proposed molecular targets for CBD is the serotonin receptor 5-HT1A. Russo et al, (2005)14 showed that in heterologous cells expressing the 5-HT1A receptor, CBD produced a dose-dependent displacement of [3H]8-OH-DPAT binding, a selective 5-HT1A agonist, and at a high dose was able to induce robust [35S]GTPγS binding, supporting an agonistic activity for CBD at this receptor.14 To further reinforce the notion that CBD is interacting with the orthosteric binding site of 5-HT1A receptors, the selective antagonist NAN-190 was used in the cAMP assay that assessed the percent inhibition of forskolin-stimulated cAMP levels in CHO cells. Both 5-HT and CBD reduced the percentage of forskolin-stimulated AMP in the cells, and this reduction was blocked by NAN-190. This suggests that NAN-190 is competing with 5-HT or CBD for the orthosteric binding site of the 5-HT1A receptor.
Behavioral studies examining the involvement of the 5-HT1A receptor found that CBD increased the percentage of time rats spent on the Elevated Plus Maze.34 This response was similar to other known anxiolytic substances, such as AEA and its analogue ACEA,35 and involved 5-HT1A activation in the dorso-lateral PAG as suggested by reversal of anxiolytic effects in the presence of a selective 5-HT1A receptor antagonist, WAY-100635.34 Additionally, a study assessing the antidepressant effects of CBD found increased rodent vertical motor activity and that this was blunted by the 5-HT1A receptor antagonist, WAY 100635.2 CBD could also potentiate the effects of 8-OH-DPAT, a selective 5-HT1A receptor agonist, in motor activity.2 This supports the involvement of the 5-HT1A receptor in the antidepressant effects of CBD.
Studies have also examined the antiallodynic effects of CBD. A study using a rat model of neuropathic pain, streptozotocin-induced diabetes, found that CBD was able to attenuate mechanical allodynia; this was blocked by WAY 100135, a selective 5-HT1A receptor antagonist, but not by AM 251 or AM 630, selective CB1 and CB2 receptor antagonists, respectively.36 Another study used a different model of neuropathic pain, paclitaxel-induced neuropathy, and showed that CBD could attenuate mechanical allodynia. The latter effect was blocked by the selective 5-HT1A receptor antagonist WAY 100635 but not by the CB₁ antagonist, SR141716, or the CB₂ antagonist, SR144528.37 Together these studies show that many of CBD’s effects are mediated through 5-HT1A receptor activation in the central and peripheral nervous system, regulating neuronal excitability and neurotransmitter release.
5. DOPAMINE RECEPTORS
CBD has been proposed as a partial agonist of D2 dopamine receptorssince it inhibits radiolabeled domperidone binding to D2 receptors with dissociation constants of 11 nmol/L for dopamine D2High receptors (dopamine D2 receptors in the high affinity state) and 2800 nmol/L for dopamine D2Low receptors (dopamine D2 receptors in the low affinity state) in rat striatal membranes.38 Through molecular modeling (Molecular mechanics energies combined with generalized Born and surface area continuum solvation, MM-GBSA) of D2 and D3 receptors in complex with CBD and haloperidol, Stark et al,39 showed that CBD might bind more favorably to D3 dopamine receptors compared to D2 receptors, and probably acts as a partial agonist at this receptor.
Although not acting directly at dopamine receptors, cannabinoids have been shown to alter dopamine signaling in the brain. The ventral hippocampus (VHipp) is responsible for transmitting emotionally relevant contextual information to the mesolimbic dopaminergic system thereby controlling the amount of dopamine being released at the ventro-tegmental area (VTA).40 Systemic or intra-VHipp injection of WIN55,212–2 (CB1 receptor agonist) was shown to increase VTA dopaminergic neuronal activity and bursting rates, decrease VTA non-dopaminergic neuronal activity, and elicit dopamine efflux directly into the nucleus accumbens shell. These effects were reversed by SR141716A (CB1 receptor antagonist).41 THC and CBD were shown to exert differential control over dopamine activity states and emotional memory processing because of their opposing effects on molecular signaling pathways underlying schizophrenia.40, 42
6. ADENOSINE RECEPTORS
CBD, alongside with THC, was shown to inhibit adenosine reuptake with an IC50 of 124 nmol/L by acting as competitive inhibitors at the equilibrative nucleotide transporter on EOC-20 microglia cells; this increases the endogenous adenosine content available for adenosine receptor activation.43 Furthermore, treatment with CBD (1 mg/kg) significantly reduced tumor necrosis factor (TNFα) in mice challenged with lipopolysaccharides (LPS); this was blocked by pre-treatment with the selective A2A adenosine receptor antagonist, ZM 241385 (10 mg/Kg, i.p.).43 The role of A2A adenosine receptors as CBD targets was confirmed by Ribeiro et al,44 who found that CBD-mediated anti-inflammatory effects were reversed by the A2A receptor antagonist, ZM 241385, in a murine model of acute lung injury.
In a different context, CBD was shown to have antiarrhythmic effects against I/R-induced arrhythmias in rats and this was blocked by the adenosine A1 receptor antagonist DPCPX,45 indicating that CBD might activate more than one type of adenosine receptor.
7. OPIOID RECEPTORS
The idea that cannabinoids might have modulatory effects at opioid receptors was initially postulated by Vaysse et al,46 where they showed that Δ9-THC decreased [3H]dihydromorphine binding to MOR due to a reduction in the number of binding sites. According to their findings, this suggests that the interaction of Δ9-THC with opioid receptors occurs in a non-competitive manner most likely acting as a negative allosteric modulator. Investigations by Kathmann et al,47 show that both THC and CBD at 30 µmol/L concentration behave as negative allosteric modulators of MOR and δ opioid receptors (DOR) since they accelerated the dissociation of [3H]-DAMGO (pEC50 = 4.67 and 4.38 for THC and CBD, respectively) and [3H]-naltrindole (pEC50 = 5.00 and 4.10 for THC and CBD, respectively) from MOR and DOR in displacement binding assays using rat brain cortical membranes. THC increased the dissociation of [3H]-DAMGO by a factor of 2; cannabidiol increased the dissociation markedly at least by a factor of 12.47
A study by Viudez-Martínez et al,48 showed that administration of CBD led to a reduction in the MOR gene expression among other genes; this led the authors to speculate that CBD might be responsible for reducing the reinforcing properties, motivation and relapse for ethanol consumption in the two-bottle choice (TBC) paradigm in mice. This experimental approach is very useful for measurement of stress-induced anhedonia in mice using sucrose as a reward stimuli in one of the bottles, as opposed to water on the other.49 It takes advantage of the fact that rodents naturally and avidly consume sweet food and selectively drink sweet drink solution when presented with a two-bottle free-choice access to both the sucrose solution and regular water. However, when exposed to stress induced models of depression, rodents failed to drink sweetened water in preference to regular water.50-52 Thus, using this model Viudez-Martínez et al,48 found that CBD (60 and 120 mg/kg/day, i.p.) reduced ethanol consumption and preference in the two-bottle choice in C57BL/6J mice. Moreover CBD significantly decreased ethanol intake and the number of effective responses in the oral ethanol self-administration. Parallel to that, they found that CBD significantly reduced Oprm1 gene expression, among other genes, leading the authors to conclude that CBD reduced the reinforcing properties, motivation and relapse for ethanol.
In this context, Hurd53 shed some light on the importance of CBD as a potential tool for the treatment of Opioid Use Disorder (OUD) pointing out that CBD is not rewarding54 and as such has limited misuse potential. Moreover CBD has remarkable positive effects on the treatment of anxiety55 and sleep disorders,56 major behavioral features of drug addiction, as well as a neuroprotective effect57 making it safe to be used at high doses for the treatment of a variety of conditions.58 With this pharmacological profile, CBD could provide a strong alternative treatment to inhibit drug-seeking behavior and curb the current opioid abuse and misuse crisis that strikes the United States and other countries.
Due to its modulatory activity over the endocannabinoid system, the close interactions between the cannabinoid and the opioid systems, its anxiolytic properties and lack of psychostimulant effects, CBD could be a powerful tool to be used in drug abuse treatments and withdrawal syndrome. For a more comprehensive review on the potential of CBD in the treatment of drug addiction, see Hurd et al (2015).59
8. ION CHANNELS
A proposed molecular target for CBD is the Transient Receptor Potential Vanilloid 1 (TRPV1) receptor (also known as VR1 receptor). A study by Bisogno et al,15 showed that CBD can displace capsaicin from the TRPV1 receptor and increase intracellular Ca2+ levels to the same extent as the full agonist capsaicin in heterologous cells overexpressing TRVR1 suggesting that it functions as an agonist of this receptor.
TRP channels belonging to subfamily V type 2 (TRPV2) and subfamily A type 1 (TRPA1) have also been implicated as potential targets of CBD in modulating neuronal hyperactivity.60 Electroencephalographic (EEG) evaluation of brain activity showed that 60 mg/kg CBD had anticonvulsant effects in a mice model of seizure induction.61 Interestingly, CBD increased seizure latency and reduced seizure duration when injected intraperitoneally, and these effects were reversed by SB 366791, AM 251 and AM 630, selective antagonists of the TRPV1, CB1, and CB2 receptors, respectively.61 This suggests an involvement of additional targets beyond the TRPV1 channel receptors, such as the endocannabinoid system, in the anticonvulsant and anti-epileptic effects of CBD.61
In a study using multiple models of cocaine self-administration, researchers evaluated the effects of a wide range of cocaine (0.031, 0.0625, 0.125, 0.25, 0.5, and 1 mg/kg/infusion) and CBD (3,10, 20, and 40 mg/kg, i.p.) doses on cocaine mediated reward behavior. Using different protocols of cocaine administration, such as the fixed ratio 1 (FR1 – cocaine reinforcement given after 1 attempt of self-administration) or the progressive ratio (PR – increasing response requirement for cocaine delivery over successive attempts of self-administration) schedule of reinforcement, coupled with in vivo microdialysis with high-performance liquid chromatography (HPLC) assays to evaluate brain dopamine levels, scientist showed that systemic administration of 20 mg/kg CBD dose-dependently inhibited cocaine self-administration; this was blocked by AM 630, WAY100135, and capsazepine (selective CB2, 5-HT1A, and TRPV1 receptor antagonists, respectively) demonstrating that targets beyond TRPV1 enable CBD effects.62 Furthermore, they showed that CBD given at the dose of 20 mg/kg attenuates cocaine-induced dopamine in the nucleus accumbens, which suggests that CBD plays an important role in controlling brain response to cocaine and the consequent drug seeking behavior triggered by drug consumption.62
Together these studies show that CBD has modulating effects at different doses (3,10, 20, and 40 mg/kg) and routes of administration (intrapretitoneal, subcutaneous), that are mainly dependent on its agonistic activity at TRPV1 and 5-HT1A receptors.14, 15 Although both receptors are responsible for several CBD-mediated actions, other targets might also be involved in distinct effects attributed to CBD and need to be further investigated.
In addition to TRPV chaanels, CBD has also been shown to engage sodium and calcium channels. CBD inhibits hNav1.1-1.7 currents, with an IC50 of 1.9–3.8 μmol/L in HEK-293 cells and in iPSC neurons, due to preferential stabilization of inactivated Nav channels.63 The effects of CBD on biophysical properties such as membrane fluidity and sodium channel conductance could be responsible for its positive outcomes in the treatment of epilepsy and other hyperactivity syndromes.64 CBD has also been shown to inhibit L-type Ca2+ channels with an IC50 of 0.1 µmol/L as detected by patch-clamp techniques in rat myocites.65 Using mitochondrion-specific Ca2+ sensor (Rhod-FF, AM), it was shown that CBD reduces [Ca2+]i levels in high excitability states and increases [Ca2+]i levels in control states in hippocampal primary culture cells.66 The modulatory properties of CBD on Na+ and Ca2+ channels might have a great impact on neuronal excitability. Sodium currents in peripheral neurons are mainly responsible for sensory afferent stimuli to reach the central nervous system.67 If CBD can control part of the afferent stimuli coming from the periphery, the analgesic effects reported by Phillpot et al and Ward et al26, 68 might be due to this ability of CBD to control membrane excitability.
9. PPARγ RECEPTORS
Peroxisome proliferator-activated receptor gamma(PPARγ) is intimately related to glucose metabolism and insulin signaling in skeletal muscle and liver.69 Thiazolidinediones are insulin-sensitizing drugs that are greatly used to treat Type 2 diabetes to improve the metabolic profile of patients.70 Some thiazolidinediones such as rosiglitazole and pioglitazole, have been shown to be PPARγ agonists and stimulate the transcription of insulin and fatty acid regulating genes leading to restoration of the glycemic profile in db/db mice.71 These are obese mice due to leptin receptor knockout that have considerably higher caloric intake, hyperglycemia, dyslipidemia, and metabolic syndrome and are commonly used as models of Type 2 Diabetes and obesity.
CBD has been shown to have agonistic activities at PPARγ that might explain CBD-mediated improvements in lipid and glycemic parameters in Type 2 Diabetes.6 Blockade of PPARγ with the selective antagonist, GW9662, significantly blunted CBD effects on reactive gliosis in rat primary astroglial cultures.72 Moreover agonism of PPARγ by CBD might be an attractive therapeutic tool for Alzheimer’s disease (AD). There is a significant body of evidence showing the efficacy of PPARγ agonists, such as pioglitazole,73 in ameliorating disease-related pathology and improving learning and memory in animal models of AD. Recent clinical trials showed a significant improvement in memory and cognition in AD patients treated with rosiglitazone.74 It is important to highlight that in addition to CBD, endogenous cannabinoids such as anandamide and 2-AG can also activate PPARγ and produce anti-inflammatory responses.75
10. CONCLUSIONS AND PERSPECTIVES
CBD’s potential as a therapeutic comes from its multiple mechanisms of action. This wide range of pharmacological activity underlies the effects of CBD on anxiety, depression, pain, memory, metabolism and more. One potential novel target is GPCR heteromers, a macromolecular complex composed of at least two functional receptor units (protomers) with biochemical properties that are demonstrably different from those of its individual components. There are three criteria for G-protein heteromers in native tissues: (a) Heteromer components should colocalize and physically interact; (b) Heteromers should exhibit properties distinct from those of the protomers; (c) Heteromer disruption should lead to a loss of heteromer-specific properties.76 CBD has been reported to be an allosteric modulator of the DOR47 and heteromers between the DOR and the CB1 cannabinoid receptors have been reported.77 Furthermore, MOR has also been shown to interact with CB1 cannabinoid receptors.22, 47, 78-81 Hence, it is possible to envision that CBD could exert some of its effects via MOR-CB1 heteromers. By characterizing heteromer fingerprints, future studies could establish MOR-CB1 heteromer as a target of CBD.
While it is important to recognize the beneficial effects of CBD, it is even more important to understand that it is not a miraculous drug that can be effectively used in any given pathology or condition. While the pharmacodynamic properties are being characterized, further studies need to be undertaken to better characterize the pharmacokinetic properties of CBD, the correct dosage and routes of administration for each specific condition, advantages of coadministration with other substances (especially with morphine in the context of pain management) and whether detrimental side-effects arise from chronic treatment with CBD.
References
-
Mechoulam R. Marihuana chemistry. Science. 1970;168(3936):1159–1166. https://doi.org/10.1126/science.168.3936.1159
CrossrefCASPubMedWeb of Science®Google Scholar -
Espejo-Porras F, Fernández-Ruiz J, Pertwee RG, Mechoulam R, García C. Motor effects of the non-psychotropic phytocannabinoid cannabidiol that are mediated by 5-HT1A receptors. Neuropharmacology. 2013;75:155–163. https://doi.org/10.1016/j.neuropharm.2013.07.024
CrossrefCASPubMedWeb of Science®Google Scholar -
Shoval G, Shbiro L, Hershkovitz L, et al. Prohedonic effect of cannabidiol in a rat model of depression. Neuropsychobiology. 2016;73(2):123–129. https://doi.org/10.1159/000443890
CrossrefCASPubMedWeb of Science®Google Scholar -
Fisher T, Golan H, Schiby G, et al. In vitro and in vivo efficacy of non-psychoactive cannabidiol in neuroblastoma. Curr Oncol. 2016;23(2):S15–S22. https://doi.org/10.3747/co.23.2893
CrossrefCASPubMedWeb of Science®Google Scholar -
Juknat A, Kozela E, Kaushansky N, Mechoulam R, Vogel Z. Anti-inflammatory effects of the cannabidiol derivative dimethylheptyl-cannabidiol - studies in BV-2 microglia and encephalitogenic T cells. J Basic Clin Physiol Pharmacol. 2016;27(3):289–296. https://doi.org/10.1515/jbcpp-2015-0071
CrossrefCASPubMedGoogle Scholar -
Jadoon KA, Ratcliffe SH, Barrett DA, et al. Efficacy and safety of cannabidiol and tetrahydrocannabivarin on glycemic and lipid parameters in patients with type 2 diabetes: a randomized, double-blind, placebo-controlled, placebo-controlled, Parallel Group Pilot Study.Diabetes Care. 2016;39(10):1777–1786. https://doi.org/10.2337/dc16-0650
CrossrefCASPubMedWeb of Science®Google Scholar -
Lehmann C, Fisher NB, Tugwell B, Szczesniak A, Kelly M, Zhou J. Experimental cannabidiol treatment reduces early pancreatic inflammation in type 1 diabetes. Clin Hemorheol Microcirc. 2016;64(4):655–662. https://doi.org/10.3233/CH-168021
CrossrefCASPubMedWeb of Science®Google Scholar -
Crippa JADS, Zuardi AW, Garrido GEJ, et al. Effects of cannabidiol (CBD) on regional cerebral blood flow. Neuropsychopharmacology. 2004;29(2):417–426. https://doi.org/10.1038/sj.npp.1300340
CrossrefCASPubMedWeb of Science®Google Scholar -
Linares IM, Zuardi AW, Pereira LC, et al. Cannabidiol presents an inverted U-shaped dose-response curve in a simulated public speaking test. Rev Bras Psiquiatr. 2019;41(1):9–14. https://doi.org/10.1590/1516-4446-2017-0015
CrossrefPubMedWeb of Science®Google Scholar -
Freeman AM, Petrilli K, Lees R, et al. How does cannabidiol (CBD) influence the acute effects of delta-9-tetrahydrocannabinol (THC) in humans? A systematic review. Neurosci Biobehav Rev. 2019;107:696–712. Published online September 30, 2019. https://doi.org/10.1016/j.neubiorev.2019.09.036
CrossrefCASPubMedWeb of Science®Google Scholar -
Borges RS, Batista J, Viana RB, et al. Understanding the molecular aspects of tetrahydrocannabinol and cannabidiol as antioxidants. Molecules. 2013;18(10):12663–12674. https://doi.org/10.3390/molecules181012663
CrossrefCASPubMedWeb of Science®Google Scholar -
Hampson AJ, Grimaldi M, Lolic M, Wink D, Rosenthal R, Axelrod J. Neuroprotective antioxidants from marijuana. Ann N Y Acad Sci. 2000;899:274–282. http://www.ncbi.nlm.nih.gov/pubmed/10863546
Wiley Online LibraryCASPubMedWeb of Science®Google Scholar -
Hacke ACM, Lima D, de Costa F, et al. Probing the antioxidant activity of Δ9-tetrahydrocannabinol and cannabidiol in Cannabis sativa extracts. Analyst. 2019;144(16):4952–4961. https://doi.org/10.1039/c9an00890j
CrossrefCASPubMedWeb of Science®Google Scholar -
Russo EB, Burnett A, Hall B, Parker KK. Agonistic properties of cannabidiol at 5-HT1a receptors. Neurochem Res. 2005;30(8):1037–1043. https://doi.org/10.1007/s11064-005-6978-1
CrossrefCASPubMedWeb of Science®Google Scholar -
Bisogno T, De Petrocellis L, Mechoulam R, et al. Molecular targets for cannabidiol and its synthetic analogues: effect on vanilloid VR1 receptors and on the cellular uptake and enzymatic hydrolysis of anandamide. Br J Pharmacol. 2001;134(4):845–852. https://doi.org/10.1038/sj.bjp.0704327
Wiley Online LibraryCASPubMedWeb of Science®Google Scholar -
Campos AC, Moreira FA, Gomes FV, del Bel EA, Guimarães FS. Multiple mechanisms involved in the large-spectrum therapeutic potential of cannabidiol in psychiatric disorders. Philos Trans R Soc B Biol Sci. 2012;367(1607):3364–3378. https://doi.org/10.1098/rstb.2011.0389
CrossrefCASPubMedWeb of Science®Google Scholar -
Di Marzo V, Bifulco M, De Petrocellis L. The endocannabinoid system and its therapeutic exploitation. Nat Rev Drug Discov. 2004;3(9):771–784. https://doi.org/10.1038/nrd1495
CrossrefCASPubMedWeb of Science®Google Scholar -
Castillo PE, Younts TJ, Chávez AE, Hashimotodani Y. Endocannabinoid signaling and synaptic function. Neuron. 2012;76(1):70–81. https://doi.org/10.1016/j.neuron.2012.09.020
CrossrefCASPubMedWeb of Science®Google Scholar -
MacLennan SJ, Reynen PH, Kwan J, Bonhaus DW. Evidence for inverse agonism of SR141716A at human recombinant cannabinoid CB1 and CB2 receptors. Br J Pharmacol. 1998;124(4):619–622. https://doi.org/10.1038/sj.bjp.0701915
Wiley Online LibraryCASPubMedWeb of Science®Google Scholar -
Pertwee RG. The diverse CB1 and CB2 receptor pharmacology of three plant cannabinoids: delta9-tetrahydrocannabinol, cannabidiol and delta9-tetrahydrocannabivarin. Br J Pharmacol. 2008;153(2):199–215. https://doi.org/10.1038/sj.bjp.0707442
Wiley Online LibraryCASPubMedWeb of Science®Google Scholar -
Laprairie RB, Bagher AM, Kelly MEM, Denovan-Wright EM. Cannabidiol is a negative allosteric modulator of the cannabinoid CB1 receptor. Br J Pharmacol. 2015;172(20):4790–4805. https://doi.org/10.1111/bph.13250
Wiley Online LibraryCASPubMedWeb of Science®Google Scholar -
Thomas A, Baillie GL, Phillips AM, Razdan RK, Ross RA, Pertwee RG. Cannabidiol displays unexpectedly high potency as an antagonist of CB1 and CB2 receptor agonists in vitro. Br J Pharmacol. 2007;150(5):613–623. https://doi.org/10.1038/sj.bjp.0707133
Wiley Online LibraryCASPubMedWeb of Science®Google Scholar -
Pertwee RG, Ross RA, Craib SJ, Thomas A. (-)-Cannabidiol antagonizes cannabinoid receptor agonists and noradrenaline in the mouse vas deferens. Eur J Pharmacol. 2002;456(1–3):99–106. https://doi.org/10.1016/s0014-2999(02)02624-9
CrossrefCASPubMedWeb of Science®Google Scholar -
Hudson R, Renard J, Norris C, Rushlow WJ, Laviolette SR. Cannabidiol counteracts the psychotropic side-effects of Δ-9-tetrahydrocannabinol in the ventral hippocampus through bidirectional control of ERK1-2 phosphorylation. J Neurosci. 2019;39(44):8762–8777. https://doi.org/10.1523/JNEUROSCI.0708-19.2019
CrossrefPubMedWeb of Science®Google Scholar -
Maione S, Piscitelli F, Gatta L, et al. Non-psychoactive cannabinoids modulate the descending pathway of antinociception in anaesthetized rats through several mechanisms of action. Br J Pharmacol. 2011;162(3):584–596. https://doi.org/10.1111/j.1476-5381.2010.01063.x
Wiley Online LibraryCASPubMedWeb of Science®Google Scholar -
Philpott HT, OʼBrien M, McDougall JJ. Attenuation of early phase inflammation by cannabidiol prevents pain and nerve damage in rat osteoarthritis. Pain. 2017;158(12):2442–2451. https://doi.org/10.1097/j.pain.0000000000001052
CrossrefCASPubMedWeb of Science®Google Scholar -
Petrosino S, Verde R, Vaia M, Allarà M, Iuvone T, Di Marzo V. Anti-inflammatory properties of cannabidiol, a nonpsychotropic cannabinoid, in experimental allergic contact dermatitis. J Pharmacol Exp Ther. 2018;365(3):652–663. https://doi.org/10.1124/jpet.117.244368
CrossrefCASPubMedWeb of Science®Google Scholar -
Couch DG, Tasker C, Theophilidou E, Lund JN, O’Sullivan SE. Cannabidiol and palmitoylethanolamide are anti-inflammatory in the acutely inflamed human colon. Clin Sci. 2017;131(21):2611–2626. https://doi.org/10.1042/CS20171288
CrossrefCASWeb of Science®Google Scholar -
Massi P, Valenti M, Vaccani A, et al. 5-Lipoxygenase and anandamide hydrolase (FAAH) mediate the antitumor activity of cannabidiol, a non-psychoactive cannabinoid. J Neurochem. 2008;104(4):1091–1100. https://doi.org/10.1111/j.1471-4159.2007.05073.x
Wiley Online LibraryCASPubMedWeb of Science®Google Scholar -
Ross RA. The enigmatic pharmacology of GPR55. Trends Pharmacol Sci. 2009;30(3):156–163. https://doi.org/10.1016/j.tips.2008.12.004
CrossrefCASPubMedWeb of Science®Google Scholar -
Whyte LS, Ryberg E, Sims NA, et al. The putative cannabinoid receptor GPR55 affects osteoclast function in vitro and bone mass in vivo. Proc Natl Acad Sci USA. 2009;106(38):16511–16516. https://doi.org/10.1073/pnas.0902743106
CrossrefCASPubMedWeb of Science®Google Scholar -
Adams MD, Earnhardt JT, Martin BR, Harris LS, Dewey WL, Razdan RK. A cannabinoid with cardiovascular activity but no overt behavioral effects. Experientia. 1977;33(9):1204–1205. https://doi.org/10.1007/bf01922330
CrossrefCASPubMedGoogle Scholar -
Celorrio M, Rojo-Bustamante E, Fernández-Suárez D, et al. GPR55: a therapeutic target for Parkinson’s disease? Neuropharmacology. 2017;125:319–332. https://doi.org/10.1016/j.neuropharm.2017.08.017
CrossrefCASPubMedWeb of Science®Google Scholar -
Campos AC, Guimarães FS. Involvement of 5HT1A receptors in the anxiolytic-like effects of cannabidiol injected into the dorsolateral periaqueductal gray of rats. Psychopharmacology. 2008;199(2):223–230. https://doi.org/10.1007/s00213-008-1168-x
CrossrefCASPubMedWeb of Science®Google Scholar -
Moreira FA, Aguiar DC, Guimarães FS. Anxiolytic-like effect of cannabinoids injected into the rat dorsolateral periaqueductal gray. Neuropharmacology. 2007;52(3):958–965. https://doi.org/10.1016/j.neuropharm.2006.10.013
CrossrefCASPubMedWeb of Science®Google Scholar -
Jesus CHA, Redivo DDB, Gasparin AT, et al. Cannabidiol attenuates mechanical allodynia in streptozotocin-induced diabetic rats via serotonergic system activation through 5-HT1A receptors. Brain Res. 2019;1715:156–164. https://doi.org/10.1016/j.brainres.2019.03.014
CrossrefCASPubMedWeb of Science®Google Scholar -
Ward SJ, McAllister SD, Kawamura R, Murase R, Neelakantan H, Walker EA. Cannabidiol inhibits paclitaxel-induced neuropathic pain through 5-HT 1A receptors without diminishing nervous system function or chemotherapy efficacy. Br J Pharmacol. 2014;171(3):636–645. https://doi.org/10.1111/bph.12439
Wiley Online LibraryCASPubMedWeb of Science®Google Scholar -
Seeman P. Cannabidiol is a partial agonist at dopamine D2High receptors, predicting its antipsychotic clinical dose. Transl Psychiatry. 2016;6(10):e920. https://doi.org/10.1038/tp.2016.195
CrossrefCASPubMedWeb of Science®Google Scholar -
Stark T, Di Bartolomeo M, Di Marco R, et al. Altered dopamine D3 receptor gene expression in MAM model of schizophrenia is reversed by peripubertal cannabidiol treatment. Biochem Pharmacol. 2020;177:114004. https://doi.org/10.1016/j.bcp.2020.114004
CrossrefCASPubMedWeb of Science®Google Scholar -
Hudson R, Rushlow W, Laviolette SR. Phytocannabinoids modulate emotional memory processing through interactions with the ventral hippocampus and mesolimbic dopamine system: implications for neuropsychiatric pathology. Psychopharmacology. 2018;235(2):447–458. https://doi.org/10.1007/s00213-017-4766-7
CrossrefCASPubMedWeb of Science®Google Scholar -
Cheer JF, Wassum KM, Heien MLAV, Phillips PEM, Wightman RM. Cannabinoids enhance subsecond dopamine release in the nucleus accumbens of awake rats. J Neurosci. 2004;24(18):4393–4400. https://doi.org/10.1523/JNEUROSCI.0529-04.2004
CrossrefCASPubMedWeb of Science®Google Scholar -
Bhattacharyya S, Morrison PD, Fusar-Poli P, et al. Opposite effects of delta-9-tetrahydrocannabinol and cannabidiol on human brain function and psychopathology. Neuropsychopharmacology. 2010;35(3):764–774. https://doi.org/10.1038/npp.2009.184
CrossrefCASPubMedWeb of Science®Google Scholar -
Carrier EJ, Auchampach JA, Hillard CJ. Inhibition of an equilibrative nucleoside transporter by cannabidiol: a mechanism of cannabinoid immunosuppression. Proc Natl Acad Sci USA. 2006;103(20):7895–7900. https://doi.org/10.1073/pnas.0511232103
CrossrefCASPubMedWeb of Science®Google Scholar -
Ribeiro A, Ferraz-de-Paula V, Pinheiro ML, et al. Cannabidiol, a non-psychotropic plant-derived cannabinoid, decreases inflammation in a murine model of acute lung injury: role for the adenosine A2A receptor. Eur J Pharmacol. 2012;678(1–3):78–85. https://doi.org/10.1016/j.ejphar.2011.12.043
CrossrefCASPubMedWeb of Science®Google Scholar -
Gonca E, Darıcı F. The effect of cannabidiol on ischemia/reperfusion-induced ventricular arrhythmias: the role of adenosine A1 receptors. J Cardiovasc Pharmacol Ther. 2015;20(1):76–83. https://doi.org/10.1177/1074248414532013
CrossrefCASPubMedWeb of Science®Google Scholar -
Vaysse PJ, Gardner EL, Zukin RS. Modulation of rat brain opioid receptors by cannabinoids. J Pharmacol Exp Ther. 1987;241(2):534–539. http://www.ncbi.nlm.nih.gov/pubmed/3033219
CASPubMedWeb of Science®Google Scholar -
Kathmann M, Flau K, Redmer A, Tränkle C, Schlicker E. Cannabidiol is an allosteric modulator at mu- and delta-opioid receptors. Naunyn Schmiedebergs Arch Pharmacol. 2006;372(5):354–361. https://doi.org/10.1007/s00210-006-0033-x
CrossrefCASPubMedWeb of Science®Google Scholar -
Viudez-Martínez A, García-Gutiérrez MS, Navarrón CM, et al. Cannabidiol reduces ethanol consumption, motivation and relapse in mice. Addict Biol. 2018;23(1):154–164. https://doi.org/10.1111/adb.12495
Wiley Online LibraryCASPubMedWeb of Science®Google Scholar -
Liu M-Y, Yin C-Y, Zhu L-J, et al. Sucrose preference test for measurement of stress-induced anhedonia in mice. Nat Protoc. 2018;13(7):1686–1698. https://doi.org/10.1038/s41596-018-0011-z
CrossrefCASPubMedWeb of Science®Google Scholar -
Sobrian SK, Marr L, Ressman K. Prenatal cocaine and/or nicotine exposure produces depression and anxiety in aging rats. Prog Neuropsychopharmacol Biol Psychiatry. 2003;27(3):501–518. https://doi.org/10.1016/S0278-5846(03)00042-3
CrossrefCASPubMedWeb of Science®Google Scholar -
Zhou Q-G, Hu Y, Wu D-L, et al. Hippocampal telomerase is involved in the modulation of depressive behaviors. J Neurosci. 2011;31(34):12258–12269. https://doi.org/10.1523/JNEUROSCI.0805-11.2011
CrossrefCASPubMedWeb of Science®Google Scholar -
Goshen I, Kreisel T, Ben-Menachem-Zidon O, et al. Brain interleukin-1 mediates chronic stress-induced depression in mice via adrenocortical activation and hippocampal neurogenesis suppression. Mol Psychiatry. 2008;13(7):717–728. https://doi.org/10.1038/sj.mp.4002055
CrossrefCASPubMedWeb of Science®Google Scholar -
Hurd YL. Cannabidiol: swinging the marijuana pendulum from “Weed” to medication to treat the opioid epidemic. Trends Neurosci. 2017;40(3):124–127. https://doi.org/10.1016/j.tins.2016.12.006
CrossrefCASPubMedWeb of Science®Google Scholar -
Katsidoni V, Anagnostou I, Panagis G. Cannabidiol inhibits the reward-facilitating effect of morphine: involvement of 5-HT1A receptors in the dorsal raphe nucleus. Addict Biol. 2013;18(2):286–296. https://doi.org/10.1111/j.1369-1600.2012.00483.x
Wiley Online LibraryCASPubMedWeb of Science®Google Scholar -
Blessing EM, Steenkamp MM, Manzanares J, Marmar CR. Cannabidiol as a potential treatment for anxiety disorders. Neurotherapeutics. 2015;12(4):825–836. https://doi.org/10.1007/s13311-015-0387-1
CrossrefCASPubMedWeb of Science®Google Scholar -
Shannon S, Lewis N, Lee H, Hughes S. Cannabidiol in anxiety and sleep: a large case series. Perm J. 2019;23:18–41. https://doi.org/10.7812/TPP/18-041
CrossrefPubMedGoogle Scholar -
Machado Bergamaschi M, Helena Costa Queiroz R, Waldo Zuardi A, Alexandre S, Crippa J. Safety and side effects of cannabidiol, a Cannabis sativa constituent. Curr Drug Saf. 2011;6(4):237–249. https://doi.org/10.2174/157488611798280924
CrossrefPubMedGoogle Scholar -
Iffland K, Grotenhermen F. An update on safety and side effects of cannabidiol: a review of clinical data and relevant animal studies. Cannabis Cannabinoid Res. 2017;2(1):139–154. https://doi.org/10.1089/can.2016.0034
CrossrefCASPubMedGoogle Scholar -
Hurd YL, Yoon M, Manini AF, et al. Early phase in the development of cannabidiol as a treatment for addiction: opioid relapse takes initial center stage. Neurotherapeutics. 2015;12(4):807–815. https://doi.org/10.1007/s13311-015-0373-7
CrossrefCASPubMedWeb of Science®Google Scholar -
Iannotti FA, Hill CL, Leo A, et al. Nonpsychotropic plant cannabinoids, cannabidivarin (CBDV) and cannabidiol (CBD), activate and desensitize transient receptor potential vanilloid 1 (TRPV1) channels in vitro: potential for the treatment of neuronal hyperexcitability. ACS Chem Neurosci. 2014;5(11):1131–1141. https://doi.org/10.1021/cn5000524
CrossrefCASPubMedWeb of Science®Google Scholar -
Vilela LR, Lima IV, Kunsch ÉB, et al. Anticonvulsant effect of cannabidiol in the pentylenetetrazole model: pharmacological mechanisms, electroencephalographic profile, and brain cytokine levels. Epilepsy Behav. 2017;75:29–35. https://doi.org/10.1016/j.yebeh.2017.07.014
CrossrefPubMedWeb of Science®Google Scholar -
Galaj E, Bi G-H, Yang H-J, Xi Z-X. Cannabidiol attenuates the rewarding effects of cocaine in rats by CB2, 5-HT1A and TRPV1 receptor mechanisms. Neuropharmacology. 2020;167:107740. https://doi.org/10.1016/j.neuropharm.2019.107740
CrossrefCASPubMedWeb of Science®Google Scholar -
Ghovanloo M-R, Shuart NG, Mezeyova J, Dean RA, Ruben PC, Goodchild SJ. Inhibitory effects of cannabidiol on voltage-dependent sodium currents. J Biol Chem. 2018;293(43):16546–16558. https://doi.org/10.1074/JBC.RA118.004929
CrossrefCASPubMedWeb of Science®Google Scholar -
Gaston TE, Szaflarski JP. Cannabis for the treatment of epilepsy: an update. Curr Neurol Neurosci Rep. 2018;18(11):73. https://doi.org/10.1007/s11910-018-0882-y
CrossrefPubMedWeb of Science®Google Scholar -
Ali RM, Al Kury LT, Yang K-HS, et al. Effects of cannabidiol on contractions and calcium signaling in rat ventricular myocytes. Cell Calcium. 2015;57(4):290–299. https://doi.org/10.1016/j.ceca.2015.02.001
CrossrefCASPubMedWeb of Science®Google Scholar -
Ryan D, Drysdale AJ, Lafourcade C, Pertwee RG, Platt B. Cannabidiol targets mitochondria to regulate intracellular Ca2+ levels. J Neurosci. 2009;29(7):2053–2063. https://doi.org/10.1523/JNEUROSCI.4212-08.2009
CrossrefCASPubMedWeb of Science®Google Scholar -
de Lera Ruiz M, Kraus RL. Voltage-gated sodium channels: structure, function, pharmacology, and clinical indications. J Med Chem. 2015;58(18):7093–7118. https://doi.org/10.1021/jm501981g
CrossrefPubMedWeb of Science®Google Scholar -
Ward SJ, Ramirez MD, Neelakantan H, Walker EA. Cannabidiol prevents the development of cold and mechanical allodynia in paclitaxel-treated female C57Bl6 mice. Anesth Analg. 2011;113(4):947–950. https://doi.org/10.1213/ANE.0b013e3182283486
CrossrefCASPubMedWeb of Science®Google Scholar -
Janani C, Ranjitha Kumari BD. PPAR gamma gene – a review. Diabetes Metab Syndr Clin Res Rev. 2015;9(1):46–50. https://doi.org/10.1016/j.dsx.2014.09.015
CrossrefCASPubMedWeb of Science®Google Scholar -
Mooradian AD, Chehade J, Thurman JE. The role of thiazolidinediones in the treatment of patients with type 2 diabetes mellitus. Treat Endocrinol. 2002;1(1):13–20. https://doi.org/10.2165/00024677-200201010-00002
CrossrefPubMedGoogle Scholar -
Moore GB, Chapman H, Holder JC, et al. Differential regulation of adipocytokine mRNAs by rosiglitazone in db/db mice. Biochem Biophys Res Commun. 2001;286(4):735–741. https://doi.org/10.1006/bbrc.2001.5460
CrossrefCASPubMedWeb of Science®Google Scholar -
Esposito G, Scuderi C, Valenza M, et al. Cannabidiol reduces Aβ-induced neuroinflammation and promotes hippocampal neurogenesis through PPARγ involvement. PLoS One. 2011;6(12):e28668. https://doi.org/10.1371/journal.pone.0028668
CrossrefCASPubMedWeb of Science®Google Scholar -
Yan Q, Zhang J, Liu H, et al. Anti-inflammatory drug therapy alters beta-amyloid processing and deposition in an animal model of Alzheimer’s disease. J Neurosci. 2003;23(20):7504–7509. http://www.ncbi.nlm.nih.gov/pubmed/12930788
CrossrefCASPubMedWeb of Science®Google Scholar -
Landreth G, Jiang Q, Mandrekar S, Heneka M. PPARgamma agonists as therapeutics for the treatment of Alzheimer’s disease. Neurotherapeutics. 2008;5(3):481–489. https://doi.org/10.1016/j.nurt.2008.05.003
CrossrefCASPubMedWeb of Science®Google Scholar -
O’Sullivan SE. Cannabinoids go nuclear: evidence for activation of peroxisome proliferator-activated receptors. Br J Pharmacol. 2007;152(5):576–582. https://doi.org/10.1038/sj.bjp.0707423
Wiley Online LibraryCASPubMedWeb of Science®Google Scholar -
Gomes I, Ayoub MA, Fujita W, Jaeger WC, Pfleger KDG, Devi LA. G protein-coupled receptor heteromers. Annu Rev Pharmacol Toxicol. 2016;56(1):403–425. https://doi.org/10.1146/annurev-pharmtox-011613-135952
CrossrefCASPubMedWeb of Science®Google Scholar -
Sierra S, Gupta A, Gomes I, et al. Targeting cannabinoid 1 and delta opioid receptor heteromers alleviates chemotherapy-induced neuropathic pain. ACS Pharmacol Transl Sci. 2019;2(4):219–229. https://doi.org/10.1021/acsptsci.9b00008
CrossrefCASPubMedGoogle Scholar -
Ugur M, Derouiche L, Massotte D. Heteromerization modulates mu opioid receptor functional properties in vivo. Front Pharmacol. 2018;9:1–10. https://doi.org/10.3389/fphar.2018.01240
CrossrefPubMedWeb of Science®Google Scholar -
Manduca A, Lassalle O, Sepers M, et al. Interacting cannabinoid and opioid receptors in the nucleus accumbens core control adolescent social play. Front Behav Neurosci. 2016;10:1–17. https://doi.org/10.3389/fnbeh.2016.00211
CrossrefPubMedWeb of Science®Google Scholar -
Rios C, Gomes I, Devi LA. mu opioid and CB1 cannabinoid receptor interactions: reciprocal inhibition of receptor signaling and neuritogenesis. Br J Pharmacol. 2006;148(4):387–395. https://doi.org/10.1038/sj.bjp.0706757
Wiley Online LibraryCASPubMedWeb of Science®Google Scholar -
Hojo M, Sudo Y, Ando Y, et al. Mu-opioid receptor forms a functional heterodimer with cannabinoid CB receptor: electrophysiological and FRET assay analysis. J Pharmacol Sci. 2008;108(3):308–319. https://doi.org/10.1254/jphs.08244FP
CrossrefCASPubMedWeb of Science®Google Scholar -
Harding SD, Sharman JL, Faccenda E, et al. The IUPHAR/BPS Guide to PHARMACOLOGY in 2018: updates and expansion to encompass the new guide to IMMUNOPHARMACOLOGY. Nucleic Acids Res. 2018;46(D1):D1091–D1106. https://doi.org/10.1093/nar/gkx1121
CrossrefCASPubMedWeb of Science®Google Scholar -
Alexander SPH, Christopoulos A, Davenport AP, et al. THE CONCISE GUIDE TO PHARMACOLOGY 2019/20: G protein-coupled receptors. Br J Pharmacol. 2019;176(Suppl):S21–S141. https://doi.org/10.1111/bph.14748
Wiley Online LibraryPubMedWeb of Science®Google Scholar -
Alexander SPH, Mathie A, Peters JA, et al. THE CONCISE GUIDE TO PHARMACOLOGY 2019/20: ion channels. Br J Pharmacol. 2019;176(S1):S142–S228. https://doi.org/10.1111/bph.14749
Wiley Online LibraryCASPubMedWeb of Science®Google Scholar -
Ryberg E, Larsson N, Sjögren S, et al. The orphan receptor GPR55 is a novel cannabinoid receptor. Br J Pharmacol. 2007;152(7):1092–1101. https://doi.org/10.1038/sj.bjp.0707460
Wiley Online LibraryCASPubMedWeb of Science®Google Scholar -
Linge R, Jiménez-Sánchez L, Campa L, et al. Cannabidiol induces rapid-acting antidepressant-like effects and enhances cortical 5-HT/glutamate neurotransmission: role of 5-HT1A receptors. Neuropharmacology. 2016;103:16–26. https://doi.org/10.1016/j.neuropharm.2015.12.017
CrossrefCASPubMedWeb of Science®Google Scholar
Publisher URL: https://onlinelibrary.wiley.com/doi/abs/10.1002/prp2.682
Open URL: https://bpspubs.onlinelibrary.wiley.com/doi/pdfdirect/10.1002/prp2.682
DOI: 10.1002/prp2.682